Research Background
Photonic molecules are an emerging physical concept that mimics the formation of molecular bonds between atoms through interactions between optical microcavities. This phenomenon has excellent optical properties and has important applications in the fields of quantum optics and nonlinear optics, such as realizing quantum random number generators [1], or being used to adjust the dispersion within the microcavities to realize dark pulse optical frequency combs [2]. However, how to dynamically adjust the coupling between microcavities has been a sticking point limiting the wide application of photonic molecules.
The photonic molecular scheme based on microelectromechanical systems (MEMS) is currently attracting much attention in academia, which can control the interaction of the optical field with the waveguide by varying the distance between the microcavities [3], but this scheme imposes extremely high requirements on the preparation process; another method is to etch Bragg grating structures in the inner wall of the micro-ring, which in turn realizes the coupling between the clockwise and counterclockwise optical modes [4]. However, this scheme is difficult to achieve dynamic tuning of the coupling coefficient; in addition, the refractive index of the material can be precisely manipulated to produce periodic changes in the propagation direction to equivalently build an intracavity grating for phase matching [5], but this requires a sufficiently high pump optical power to adequately stimulate the nonlinear effect. In view of this, exploring a novel mode coupling mechanism to simplify the regulation process and realize the flexible switching of photonic molecular states is of great significance for expanding the application range of photonic molecules.
Recently, Wang Xingjun and Shu Haowen from the School of Electronics at Peking University proposed the concept of "integrated photonic molecular switch" for the first time. This work is based on the natural characteristics of multi-mode waveguide, in the microcavity bending at the fine-tuning of multiple spatial modes of coupling, only with the help of thermo-optic effect to change the refractive index of the material, can be dynamically adjusted to the coupling relationship between different spatial modes, and then realize the flexible switching of photonic molecular state. At the same time, this coupling mechanism can be realized in a very small bending size, which can achieve a large free spectral range (FSR), based on which, if the photon molecules are switched to the off state, only the fundamental mode in the microcavity can be resonated, and a very high quality factor (Q) can be achieved at the same time, thus breaking the traditional method of the two parameters of the tradeoffs for the field of microwave photonics and other areas to provide important prospects of the application.
Research Content
The research team chose a runway-type micro-ring to design the integrated micro-cavity, performed the device design on a single-crystal silicon on insulator (SOI) thin film (SOF) platform, and extended the width of the silicon waveguide to 2 micrometers to enable it to support higher-order spatial modes. Meanwhile, compared to the conventional scheme of using large Euler bends to achieve a slow-change adiabatic approximation, the team utilized Euler bends + circular arcs to construct a symmetric 180°-bent waveguide with an intentionally drastically reduced bend size to efficiently induce higher-order spatial modes within the microcavity. The geometries of the two schemes are shown in Fig. 1.
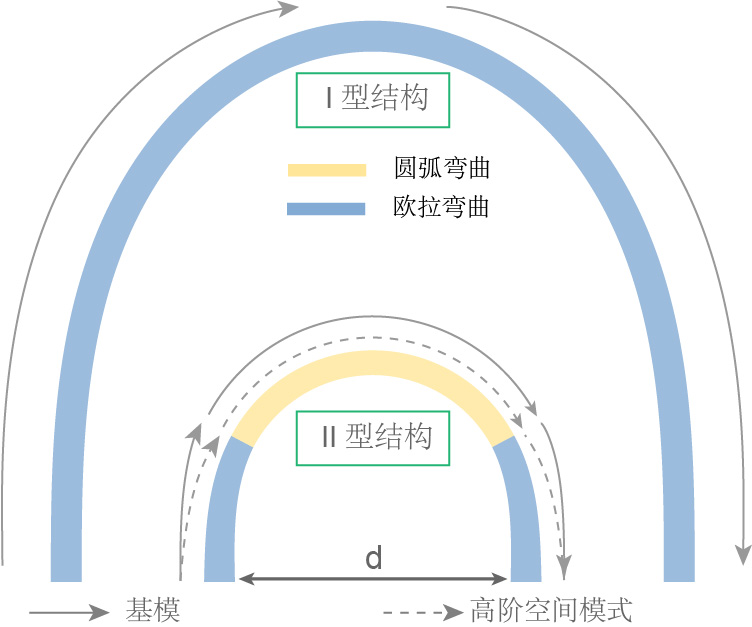
Fig 1 Schematic of two different 180° bent waveguide structures
The researchers have modified the classical coupled mode equations with reference to the rotating wave approximation, so as to analyze the coupling law under this problem more effectively. In addition, the researchers also introduced the concept of "reciprocity point" based on the distribution of the optical field intensity in the curved waveguide. The position of the reciprocity point in the curved waveguide determines the proportion of each mode at the output port, which can be calculated by the above equations. When there is only a fundamental mode at the output port (as shown in Fig. 2 i & iii), only this mode can form a resonance in the whole microcavity, and at this time the photon molecules are in the closed state; when the output port is a combination of fundamental and higher-order modes (as shown in Fig. 2 ii), the photon molecules are in the open state at this time, and according to the different occupancy ratios, the frequency intervals of the spectra that produce the splitting are also different. Therefore, the point of mutual anisotropy can be used as a "switch" to switch the physical state of the photon molecules in the microcavity, and empower a number of different fields to realize transformative breakthroughs.
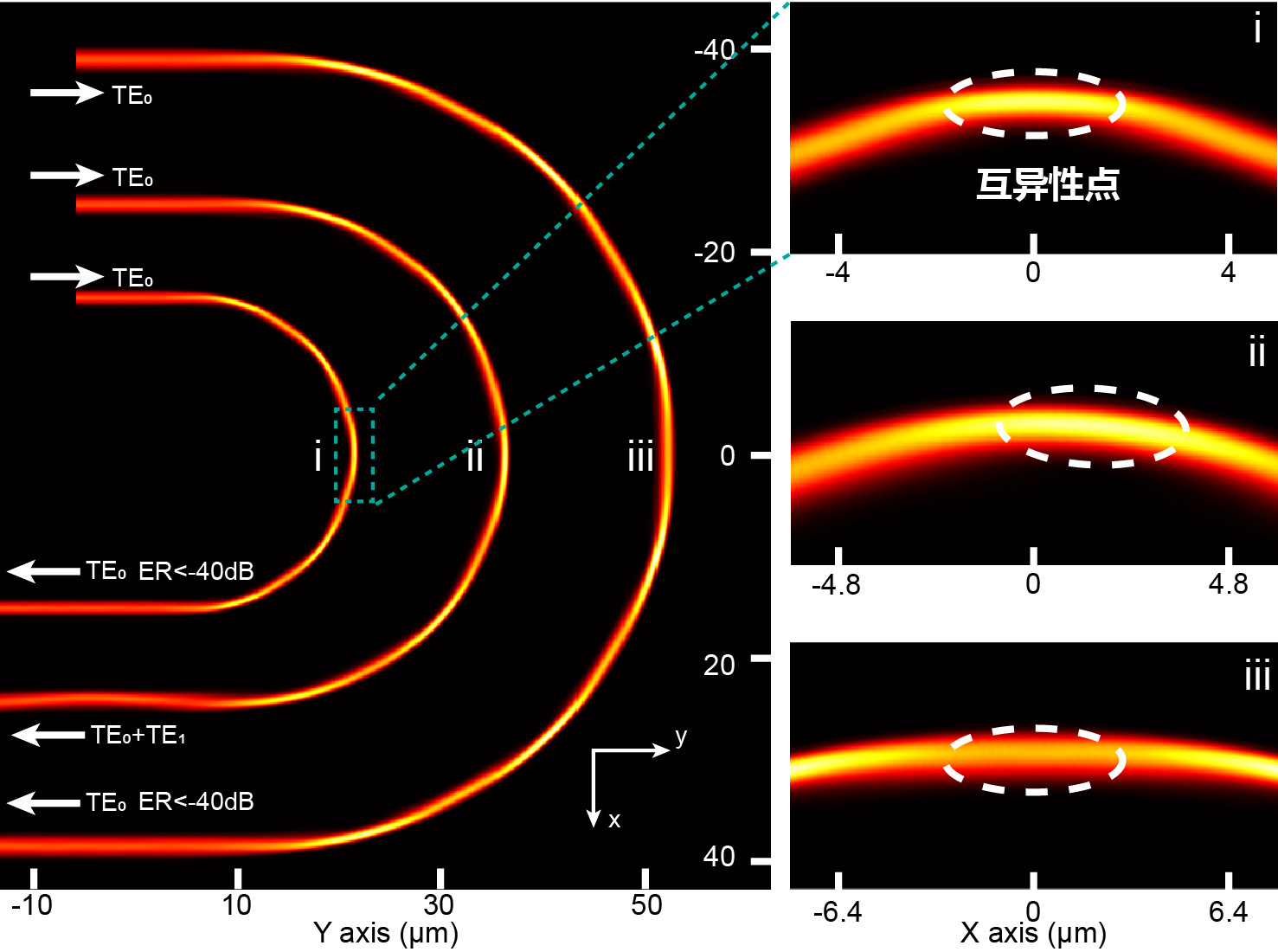
Fig 2 Three-dimensional electromagnetic field simulation results of three different bending structures
Application in open state: splitting is flexible and adjustable
The researchers changed the refractive index of the material in the localized region by placing on-chip thermal electrodes at the straight waveguide and at the curved waveguide of the micro-ring (shown in Fig. 3(a)). When a voltage is applied to the thermal electrode ① at the straight waveguide, the phase difference between the modes will be changed due to the different response of the modes to heat, and the resonance peaks formed by the two modes will be close to each other in the optical frequency domain and produce the splitting phenomenon (shown as the blue line in Fig. 3(b)), and a symmetric splitting bimodal peak will be produced when the two resonance peaks are equal in frequency. When a voltage is applied to the thermal electrode ② ③ at the curved waveguide, the change of the refractive index of the material will change the position of the mutual anisotropy point, which will change the coupling strength of the two resonance peaks, resulting in cleavage double peaks with different frequency spacings (shown as the blue line in Fig. 3 (c)).
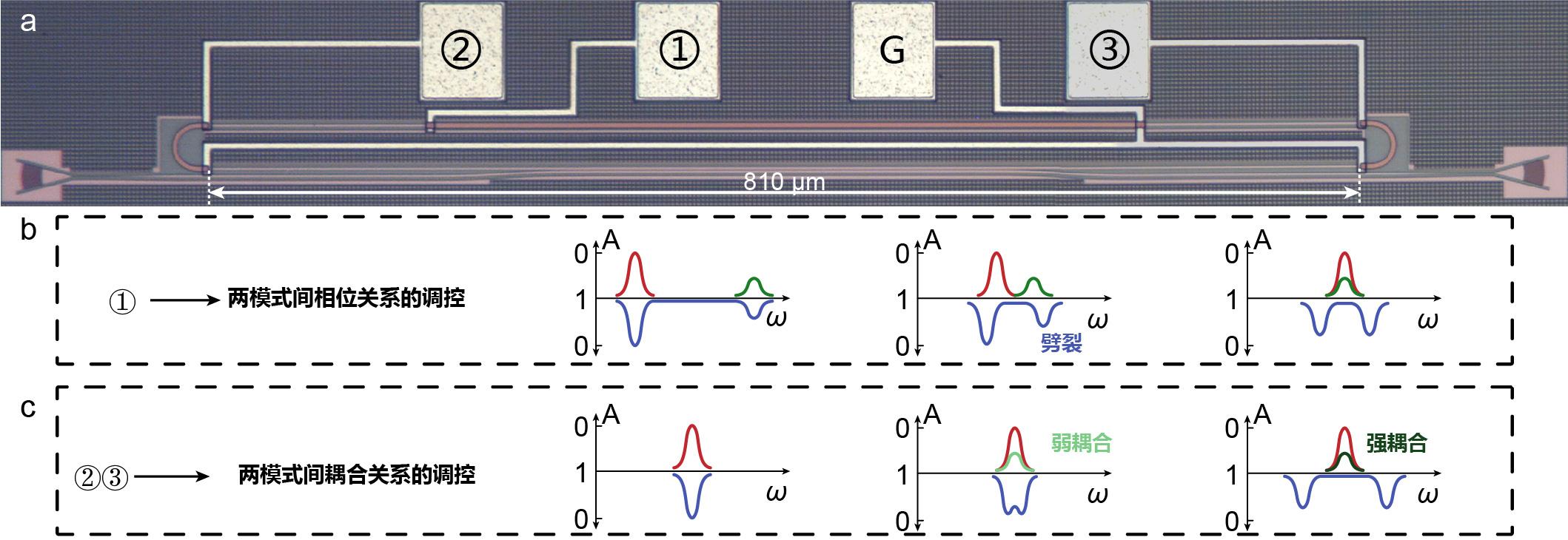
Fig. 3 a, Optical microscope diagram of the microcycle; b, Schematic diagram of the dynamic regulation mechanism
Off-state applications: ultra-wideband microwave/millimeter wave generation and processing
Due to the extremely small size of the micro-ring bending waveguide, the micro-ring has an extremely large FSR in the frequency domain, while the fundamental mode enables zero crosstalk and low-loss transmission inside the ring when the photon molecules are in the off state. On this basis, the researchers achieved the largest product of intrinsic Q and FSR based on the standard silicon optical process, substantially outperforming other work on silicon-based platforms (shown in Fig. 4).
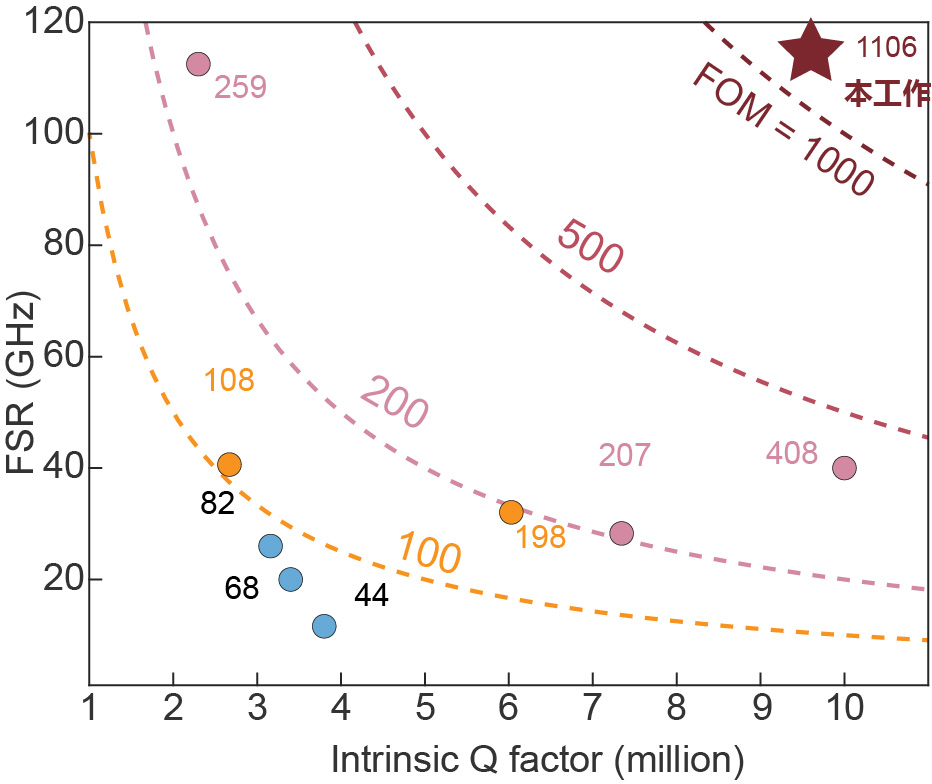
Fig. 4 Summary of the performance of the silicon-based platform micro-ring
As a key component in the microwave photonics link, the micro-ring is capable of providing extremely fine filtering over an ultra-wide frequency spectrum. The microwave photonic filter based on this micro-ring is able to achieve continuous tuning up to 58 GHz in the L, S, C band to U band range, with a 3 dB filter linewidth as narrow as 32 MHz (shown in Fig. 5a). This result actually covers only half of the FSR due to the use of a phase-modulation trans-emphasis (PM-IM) scheme. In the future, continuous and uninterrupted filtering up to 116 GHz could be achieved in combination with higher bandwidth modulators and detectors. On this basis, the research team successfully completed the experimental validation of an optoelectronic oscillator (OEO) with the help of this micro-ring, realizing a tunable microwave source with a center frequency ranging from sub-6G to 50 GHz (shown in Fig. 5b). This filter and OEO represent the widest frequency band and finest longitudinal mode filtering achieved internationally based on integrated silicon-based devices.
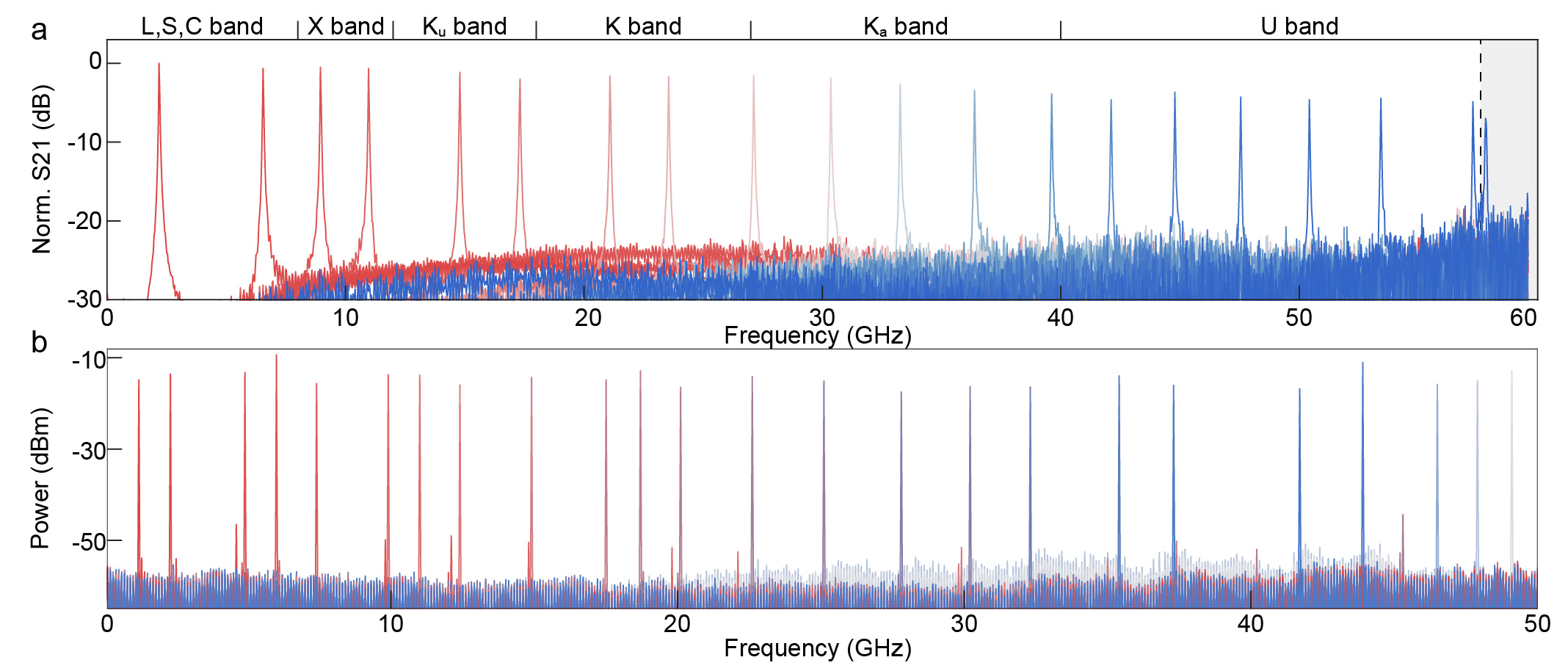
Fig. 5 Results of the filter (a) and OEO (b) realized based on this device
Prof. Xingjun Wang pointed out, "Although we did not specifically emphasize it in the paper, the most promising application of this research is in the direction of airborne, satellite and other areas with extremely stringent requirements on the weight of equipment. This is because our chip is able to replace the traditional large RF switch arrays and realize cross-band signal generation and processing with only a single component."
The article was published in Light: Science & Applications, one of the top international optical journals, under the title of "Versatile photonic molecule switch in multimode microresonators". This research work was supported by the Key Research and Development Program. Zihan Tao, a PhD student at the School of Electronics, Peking University, was the first author of the paper, Prof. Xingjun Wang and Haowen Shu were the corresponding authors.
References:
1. Okawachi Y, Kim B Y, Zhao Y, et al. Dynamic control of photon lifetime for quantum random number generation[J]. Optica, 2021, 8(11): 1458-1461.
2. Helgason Ó B, Arteaga-Sierra F R, Ye Z, et al. Dissipative solitons in photonic molecules[J]. Nature Photonics, 2021, 15(4): 305-310.
3. Siegle T, Schierle S, Kraemmer S, et al. Photonic molecules with a tunable inter-cavity gap[J]. Light: Science & Applications, 2017, 6(3): e16224-e16224.
4. Lu X, Rao A, Moille G, et al. Universal frequency engineering tool for microcavity nonlinear optics: multiple selective mode splitting of whispering-gallery resonances[J]. Photonics research, 2020, 8(11): 1676-1686.
5. Hu J, Nitiss E, He J, et al. Photo-induced cascaded harmonic and comb generation in silicon nitride microresonators[J]. Science Advances, 2022, 8(50): eadd8252.
Link to the original paper:
https://doi.org/10.1038/s41377-024-01399-0