On September 10, 2024, the Center team, in collaboration with Chang Lin's team at the School of Electronics, published the research article "High-coherence parallelization in integrated photonics" online in Nature Communications. This study demonstrates a novel low-cost, highly coherent integrated parallel source generation scheme. By combining a self-injection-locked optical frequency comb and an array of injection-locked lasers, a record on-chip 60 dB gain at near-zero noise figure is achieved. In addition, by combining this technology with silicon optical coherent modules, a total data rate of more than 60 Tbit/s is achieved and phase-dependent digital signal processing (DSP) overhead is reduced by 99.99999 percent. This research paves the way for the realization of large-scale, high-performance, high-capacity coherent optical systems, which are expected to solve the inter-chip and inter-data-centre interconnection problems brought about by the explosive growth in traffic demand, and bring new hope for the development of future optical communication networks.
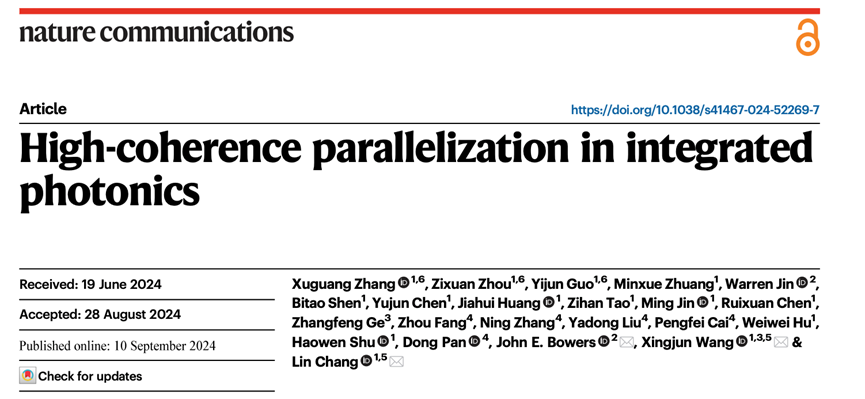
Screenshot of the article
Due to the ability to simultaneously manipulate the amplitude and phase of light, coherent optics has become one of the key trends in integrated optics over the past decade or so, bringing unlimited possibilities for a wide range of applications, including optical communications, sensing, and quantum information. However, traditional schemes for building coherent systems in integrated optics require significant costs in terms of hardware and power consumption, with a central challenge being the light source. To date, there is no method that simultaneously achieves highly parallel, coherent, and high-power integrated light sources. Although Group III-V DFB lasers are widely used for their excellent output optical power and electro-optical conversion efficiency (WPE), their intrinsic linewidths are usually at the 100 kHz level, which makes it difficult to satisfy the coherence requirements in many applications. To improve coherence, Group III-V lasers are often combined with high-quality factor microcavities to effectively reduce the linewidth to below 1 kHz and generate optical frequency combs. However, this approach sacrifices power and WPE per channel. The microcavity optical frequency comb technology that has emerged in recent years enables the generation of multiple wavelength light sources from a single device, but its power per channel is typically below -10 dBm. As a result, amplification with gains in excess of 30 dB is typically required in systems, which poses a challenge for integrated erbium-doped fiber amplifiers and semiconductor optical amplifiers, and both of which inevitably introduce additional noise.
Another challenge faced by integrated coherent systems is the significant DSP overhead. Compared to optical communication systems emphasizing direct detection, coherent detection requires more complex DSP to accurately recover frequency and phase information, which substantially increases the power consumption budget. As a result, dedicated chips are typically needed for processing. To deploy advanced coherent communication systems in next-generation data centers, DSP chips must adopt 3 nm CMOS technology to reduce power consumption. Moreover, the complexity of DSP makes real-time data processing more challenging. While methods such as laser synchronization have been proposed to reduce the reliance on DSP, these approaches often require bulky narrow-linewidth light sources and phase-locked loop technologies, significantly increasing the hardware burden of the system.
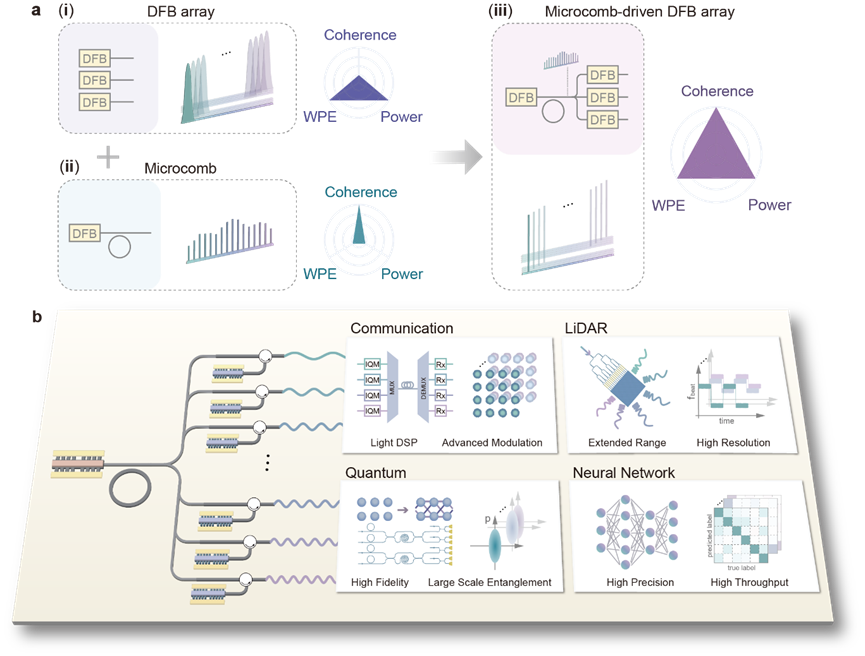
Systems based on integrated highly coherent parallel light sources
In this work, the research team demonstrated a low-cost method for achieving highly coherent and parallelized light sources in integrated optics, thereby overcoming the aforementioned challenges. Unlike improving the efficiency of coherent light sources themselves, this technique uses a highly coherent self-injection-locked optical frequency comb as a seed light to injection-lock DFB lasers. Through theoretical analysis and experimental validation, the approach effectively combines the advantages of microcavity optical frequency combs and DFB lasers, successfully achieving light sources with high coherence, high power, and high wall-plug efficiency (WPE).
Furthermore, the research team conducted parallel coherent transmission experiments using silicon photonic transceivers, demonstrating that this strategy significantly enhances the performance of integrated coherent optical communication systems. It achieved a total data transmission rate exceeding 60 Tbit/s while effectively reducing the coherent DSP overhead. This novel integrated high-coherence parallel light source approach is poised to advance coherent optical communication systems, offering great potential for coherent sinking in optical interconnects and data centers.
Professor Wang Xingjun from Peking University, Researcher Chang Lin from the School of Electronics at Peking University, and Professor John E. Bowers from the University of California, Santa Barbara, are the corresponding authors of the paper. The first authors are Zhang Xuguang, Zhou Zixuan, and Guo Yijun, PhD students at the School of Electronics, Peking University. Key collaborators include Researcher Shu Haowen, Professor Hu Weiwei, Postdocs Shen Bitao and Chen Ruixuan, PhD students Zhuang Minxue, Chen Yujun, Huang Jiahui, and Tao Zihan, as well as Dr. Jin Ming, a graduate from Peking University. Assistant Researcher Ge Zhangfeng from the Yangtze Delta Institute of Optoelectronics, Peking University, Dr. Warren Jin from the University of California, Santa Barbara, and Fang Zhou, Zhang Ning, Liu Yadong, Cai Pengfei, and Pan Dong from SiFotonics Technologies also contributed significantly. This work was conducted under the School of Electronics at Peking University and the State Key Laboratory of Advanced Optical Communication Systems and Networks.
Link to the original paper:https://www.nature.com/articles/s41467-024-52269-7